Ischaemic heart disease (IHD) is primarily caused by various combinations of three mechanisms – epicardial organic coronary stenosis attributable to atherosclerosis, epicardial coronary artery spasm and coronary microvascular dysfunction (CMD).1–3 The representative clinical manifestations of these three mechanisms are effort angina, vasospastic angina (VSA) and microvascular angina (MVA), respectively. To date, much attention has been paid to the first mechanism, epicardial organic coronary stenosis, leading to the successful developments of percutaneous coronary intervention (PCI) and coronary artery bypass grafting (CABG). However, approximately 40% of patients with obstructive coronary artery disease (CAD) still suffer from persistent/recurrent angina even after complete revascularisation with PCI and/or CABG.4 In fact, numerous patients with symptoms and evidence of myocardial ischaemia have no significant epicardial organic coronary stenosis (>50%).
Epicardial spasm and CMD, which represent typical manifestations of coronary functional abnormalities, account for the condition recently termed as ischaemia with non-obstructive coronary arteries (INOCA).5 Importantly, the prevalence of INOCA has rapidly increased, reported as reaching approximately 70% in women and 50% in men undergoing coronary angiography.6 Furthermore, to the surprise of the cardiology community, the ISCHEMIA trial convincingly demonstrated that a revascularisation strategy with PCI or CABG has no significant prognostic benefit in patients with stable CAD and proven moderate to severe myocardial ischaemia.7 These lines of evidence indicate the importance of coronary functional abnormalities in the pathogenesis and prognosis of chronic coronary syndrome, which has been described in the excellent European Association of Percutaneous Cardiovascular Interventions expert consensus document.8
Although aetiologies of impaired coronary vasomotion appear to be complex and heterogeneous, they always encompass vasodilator and vasoconstrictive properties in various combinations, where endothelial dysfunction and hypercontraction of vascular smooth muscle cells (VSMC) are substantially involved.3,9,10 Various non-invasive and invasive techniques have been developed to evaluate coronary vasomotion during the last decade.11,12 The aim of this review is to briefly summarise the current knowledge on the pathophysiology and diagnosis of coronary functional abnormalities.
Pathophysiology of Coronary Functional Abnormalities
Endothelial Dysfunction
Endothelial dysfunction has been shown to be a key mediator in the pathogenesis of coronary functional abnormalities.3,13,14 The endothelium plays pivotal roles in modulating the tone of underlying VSMC by synthesising and releasing endothelium-derived relaxing factors (EDRFs), including vasodilator prostaglandins, nitric oxide (NO), and endothelium-dependent hyperpolarisation (EDH) factors, as well as endothelium-derived contracting factors (Figure 1).15–17 Endothelial dysfunction is characterised by reduced production and/or action of EDRFs, serving as the hallmark of atherosclerotic cardiovascular diseases as well as one of the major pathogenetic mechanisms of CMD. It is important to note that these EDRFs regulate vascular tone in a distinct vessel size-dependent manner (Figure 1).

Endothelium-derived NO mainly mediates vasodilatation of relatively large, conduit vessels (e.g. epicardial coronary arteries), while EDH factors-mediated responses are the predominant mechanisms of endothelium-dependent vasodilatation of resistance arteries (e.g. coronary microvessels).16,17 Experimental studies have demonstrated that inhibition of endothelial NO production can aggravate myocardial hypoperfusion distal to an epicardial organic stenosis and that reduced NO bioavailability results in attenuation of endothelium-dependent vasodilatation.16 On the other hand, endothelium-derived H2O2, which exerts various cardioprotective effects, such as metabolic coronary dilatation, coronary autoregulation, and myocardial protection against reperfusion injury, is a major EDH factor in various vascular beds including human coronary arteries.3,17–19 Thus, it is conceivable that impaired H2O2/EDH factor-mediated vasodilatation is involved in the pathogenesis of CMD.1,17 Indeed, our recent study revealed that both NO- and EDH-derived vasodilatations are prominently impaired in patients with MVA.14
Rho-kinase as a Key Molecule of Coronary Artery Spasm
Coronary artery spasm causes a primary reduction in coronary blood flow (CBF), resulting in the abrupt development of myocardial ischaemia. It can develop in both epicardial coronary arteries and coronary microvessels. Since coronary spasm can be induced by a variety of stimuli with different mechanisms of action (even in the same patient), it is not caused by abnormalities of specific agonist–receptor interaction but an enhanced reactivity (hyperreactivity) of the vessel to a generalised stimulus.20 Reduced availability of endogenous vasodilator substances and VSMC hypersensitivity may contribute to vascular hyperreactivity leading to the onset of coronary spasm, whereas the role of endothelial dysfunction may be minimal.3 Evidence for the primary role of VSMC hypercontraction but not endothelial dysfunction in the pathogenesis of coronary artery spasm is summarised in Table 1.3
Accumulated evidence proves that a major mechanism of VSMC hypersensitivity is caused by enhanced Rho-kinase activity, an enzyme that controls contraction and relaxation of VSMC independently of intracellular Ca2+ concentration (Figure 1).21 Activated Rho-kinase enhances myosin light chain phosphorylation through inhibition of the myosin-binding subunit of myosin phosphatase, leading to VSMC hypercontraction. Importantly, the Rho-kinase inhibitor fasudil has been shown to prevent acetylcholine (ACh)-induced coronary artery spasm in patients with VSA, confirming that Rho/Rho-kinase pathway plays a central role in the pathophysiology of coronary spasm.22 It is known that various inflammatory stimuli, including angiotensin II and interleukin-1β, upregulate the expression and activity of Rho-kinase in VSMCs.3 Intriguingly, oestrogen has an inhibitory effect on inflammation-induced Rho-kinase up-regulation, which may partly account for the increased incidence of atherosclerotic and vasospastic disorders in postmenopausal women.5,23
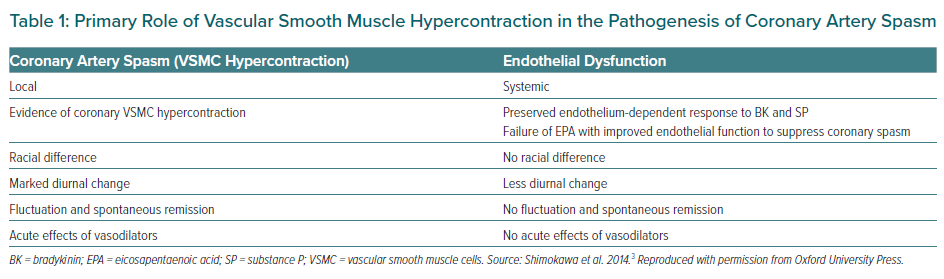
Diagnosis of Coronary Functional Abnormalities
Clinical Importance of the Evaluation of Epicardial Coronary Vasoreactivity
Coronary artery spasm plays an important role in the pathogenesis of a wide variety of IHD, including VSA, variant angina, acute MI, sudden cardiac death and intractable angina after successful coronary stent implantation.24–26 In particular, VSA is the centre of entity among disorders that represent hyperreactivity of epicardial coronary arteries to vasoconstrictor stimuli. Thus, it is important to make a correct diagnosis of VSA and evaluate epicardial coronary vasoreactivity.
Clinical Definition and Diagnostic Criteria of Vasospastic Angina
Definite VSA is diagnosed when ischaemic ECG changes – defined as a transient ST-segment elevation or depression of >0.1 mV, or new appearance of negative U waves in at least two contiguous leads – are documented during spontaneous angina attack.27 In cases without such diagnostic ECG ischaemic changes, definite VSA is angiographically diagnosed when transient, total, or subtotal (>90% stenosis) of a coronary artery accompanied by angina pain and ischaemic ECG changes during spasm provocation testing with ACh, ergonovine, or hyperventilation.27 The position paper from the Coronary Artery Vasomotion Disorders International Study group also refers to typical symptoms for VSA as follows: subjective symptoms often appear at rest, especially between night and early morning; exercise tolerance is markedly reduced in the morning; hyperventilation relates to the symptoms; and calcium-channel blockers (CCBs) are effective to suppress the symptoms.28
Clinical Significance of Pharmacological Spasm Provocation Testing
Intracoronary pharmacological spasm provocation testing is highly recommended for patients with suspected coronary artery spasm.27,28 In particular, high diagnostic accuracy of ACh testing for coronary spasm (90% sensitivity, 99% specificity) is universally recognised.29 Thus, invasive ACh provocation testing remains the gold standard of diagnostic approach of coronary spasm at present, although non-invasive spasm provocation testing including transthoracic echocardiography with IV ergonovine has also been proposed.30 Recent studies from Europe also revealed that pharmacological spasm provocation testing with ACh is safe and that coronary spasm plays a key role in development of a broad range of IHD in white patients as it does in Asian patients.31,32 Importantly, Japanese and German patients with VSA have similar results of ACh provocation testing in terms of distribution of spasm type and frequency of multivessel spasm.33 Moreover, risk stratification for VSA patients can be done using the findings of ACh testing. Indeed, studies have demonstrated that a mixture of focal and diffuse spasm provoked in multivessel coronary arteries strongly correlated with the occurrence of adverse events during the follow-up period, while coronary spasm induced at the site of significant organic stenosis was also associated with poor prognosis of VSA patients.34,35
The Japanese Coronary Spasm Association (JCSA) risk score could provide comprehensive risk assessment and prognostic stratification for VSA patients, which consists of seven variables, including history of out-of-hospital cardiac arrest (OHCA; 4 points), smoking, rest angina alone, organic coronary stenosis, multivessel spasm during spasm provocation testing (2 points each), ST-segment elevation during angina and β-blocker use (1 point each; Table 2).36 Among those seven variables, organic coronary stenosis and multivessel spasm could be identified by angiography and pharmacological spasm provocation testing.
Diagnostic Approaches to Sudden Cardiac Death Related to Coronary Spasm
Syncope, which is caused by ventricular tachyarrhythmias or bradycardia due to transient conduction disturbances, is an important manifestation of VSA. It is commonly preceded by anginal pain, although not in all cases. In a subgroup of survivors with OHCA, coronary spasm and silent myocardial ischaemia were identified as a likely cause of their fatal arrhythmias.37 Since VSA patients who survived OHCA are a particularly high-risk population, even in the current era with established effective therapies such as long-acting CCBs, implantation of an ICD with optimal medications may be appropriate for them.38,39 We have previously reported that the dual induction tests for coronary artery spasm and lethal ventricular arrhythmias could stratify high-risk OHCA patients and evaluate the necessity of ICD by underlying mechanisms involved.40 Among OHCA survivors without structural heart disease, provokable coronary spasm and ventricular arrhythmias were common and could also be seen in Brugada syndrome.40 Thus, patients with coronary spasm alone but not Brugada syndrome who are treated with CCBs could be regarded as a low-risk group, indicating that ICD may not be essential for them.
Diagnostic Imaging for Coronary Spasm
Cardiovascular imaging could provide additional information at cellular and molecular levels in patients with VSA. An intravascular imaging study showed that atherosclerotic changes are more common in the segment of focal spasm site as compared with diffuse spasm.41 In particular, optical coherence tomography (OCT), which is a high-resolution imaging modality, is capable of visualising not only morphological features of coronary vascular wall but also adventitial vasa vasorum formation at the spasm site.42,43 Chronic inflammatory changes in the coronary adventitia play important roles in the pathogenesis of coronary spasm through Rho-kinase activation and resultant VSMC hypercontraction.44 Indeed, OCT-delineated adventitial vasa vasorum formation was significantly enhanced at the spastic segments of VSA patients compared with those of control subjects.43,45 Coronary perivascular adipose tissue volume measured by CT coronary angiography is also increased at the spastic segment of VSA patients.46 Intriguingly, coronary perivascular adipose tissue inflammatory changes evaluated by 18F-fluorodeoxyglucose PET imaging were more extensive at the spastic coronary segments of VSA patients as compared with control subjects, and the inflammatory changes were significantly suppressed after medical treatment with CCBs.46 These imaging approaches may serve as a promising avenue for elucidation of the pathogenesis of coronary spasm in patients with VSA.
Advances in Diagnostic Approaches to Coronary Microvascular Dysfunction
CMD has emerged as a third potential mechanism of myocardial ischaemia in addition to atherosclerotic stenosis and spasm of epicardial coronary arteries.2,3 Since the presence of CMD appears to be associated with increased risk of cardiovascular events, it is important to make a correct diagnosis of CMD based on objective and functional assessments of coronary microcirculation.47 From a pathophysiological point of view, CMD represents the impairment in inherent regulatory mechanisms of coronary microvasculature for myocardial blood flow to adapt to changes in myocardial oxygen demand.48 More specifically, CMD is originated by a variable combination of impaired vasodilatation and increased vasoconstriction of coronary microvessels.48 However, in contrast with epicardial coronary arteries, coronary microvessels cannot be directly visualised in vivo with coronary angiography or intracoronary imaging devices. Thus, in the clinical setting, microvascular function must be assessed indirectly, generally through measurements of coronary blood flow regulated mainly by coronary arteriolar tone, or detection of propensity to coronary vasoconstriction.
Clinical Characteristics of Microvascular Angina Due to Coronary Microvascular Dysfunction
Patients with MVA due to CMD often have chest pain that can persist even after cessation of the activity. They have no rapid or sufficient symptom relief in response to sublingual nitroglycerin, which selectively dilates larger arteries but not arterioles or microvessels.49 Based on these clinical features of MVA, the Coronary Vasomotor Disorders International Study group has proposed diagnostic criteria for MVA (Table 3).50 Angina occurs in approximately 30–70% of patients with CMD, whereas other cardiac manifestations of CMD include exertional dyspnoea and possibly heart failure.51–53 CMD may represent an ischaemic equivalent caused by left ventricle (LV) diastolic dysfunction with an excessive rise in end-diastolic pressure leading to cardiopulmonary congestion. Indeed, coexistence of CMD and LV diastolic dysfunction is associated with a remarkably increased risk of heart failure with preserved ejection fraction (HFpEF) hospitalisation.53 Particularly in patients with hypertension and preserved systolic function, CMD as well as subclinical myocardial mechanical dysfunction predicted HF hospitalisation independently of LV remodelling severity.54
Assessment for Vasodilator Function of Coronary Microvessels
Coronary microvascular vasodilator function is usually assessed by measurement of coronary microvascular response to vasodilator stimuli. In many cases, the vasodilator capacity is often evaluated by coronary flow reserve (CFR) calculated as the ratio of CBF during maximal vasodilatation over basal CBF.11,12 CFR reflects an integrated measurement of flow throughout both large epicardial artery and coronary microvessels. Thus, without obstructive stenosis of epicardial coronary arteries, reduced CFR indicates the entity of CMD.11,12 Depending on the methodology, CFR cut-off values between ≤2.0 and ≤2.5 are indicative for impaired coronary microvascular function.55
The most widely used agent to assess coronary microvascular dilator function is adenosine. Adenosine is intravenously administered at 140 μg/kg/min, as this dose has been found to achieve maximal coronary microvascular dilatation.56 Adenosine has possible adverse effects, including bradycardia due to atrioventricular or sino-atrial node blockade and bronchoconstriction, both of which are mediated by purinergic A1 receptors. However, a relevant advantage of adenosine is its very short half-life (10 seconds) that enables rapid regression of side-effects and repetition of the test during the same session, if necessary.56 ACh is also used as a coronary microvascular vasodilator.52 However, ACh is not an ideal substance to assess endothelium-dependent vasodilator function, since it also acts directly on VSMCs, leading to vasoconstriction (Table 1).3
There is a range of non-invasive approaches for evaluation of coronary vasodilator responses, but all of them have several limitations.11 Transthoracic Doppler echocardiography (TTDE) enables us to measure coronary blood flow velocity of the distal left anterior descending artery as a surrogate for CBF with advantages of low cost and high feasibility. A reduced coronary blood flow velocity reserve index obtained by TTDE helps to identify patients with CMD and enables the risk stratification for them.57 On the other hand, considerable intra- and inter-observer variabilities (~10%) need to be taken into account when examining serial recordings obtained for assessing the effects of therapy.58
PET is a well-validated technique that can provide non-invasive, accurate, and reproducible quantification of myocardial blood flow and CFR in humans, and thus has been used for the assessment of coronary vasomotor function.48 PET also has the advantage of assessing all three coronary distributions, allowing a more accurate assessment of microvascular dysfunction, where CMD has been shown to have a heterogenous distribution over the three coronary arteries.48 Recent PET studies demonstrated that coronary vasodilator dysfunction, as defined by reduced CFR, is highly prevalent among patients with CAD, increases the severity of inducible myocardial ischaemia and subclinical myocardial injury, and identifies patients at high risk for future cardiac events.59,60
Cardiac magnetic resonance (CMR) has also been used to quantify myocardial perfusion following the injection of a gadolinium-based contrast agent. Advantages of CMR include high spatial resolution that allows transmural characterisation of myocardial blood flow, the lack of ionising radiation, and the ability to perform a comprehensive assessment of cardiovascular structure and function. In particular, CMR-derived myocardial perfusion reserve index (MPRI) is a robust semi-quantitative imaging surrogate that reflects vasodilator capacity of coronary microvessels.61 Recent studies demonstrated that a MPRI threshold of ≤1.84 has a diagnostic predictive value for CMD and that of ≤1.47 is associated with the occurrence of major adverse cardiac events.62,63
Coronary angiography combined with complementary catheter-based devices is a useful approach to examine coronary vasodilator capacity of patients with CMD.11,12 It often involves an interventional procedure where a guidewire-based assessment of CBF is performed at rest and at hyperaemia induced by pharmacological agents (e.g. adenosine).32,52,64 Although the procedure is invasive by nature and can be time-consuming, it has been shown to be safe and effective when performed by experienced interventional operators as discussed in detail later.65
Objective Documentation of Coronary Microvascular Spasm
In a sizable number of patients with angina, pressure-rate product (an index of myocardial oxygen demand) is usually comparable between at rest and at onset of attack, indicating that the decrease in CBF rather than increased myocardial oxygen consumption is a likely explanation for myocardial ischaemia.48 Primary reduction in CBF caused by coronary spasm at not only epicardial conduit arteries but also myocardial microvasculature could be attributable to angina at rest.66 Since a visualisation of coronary microvascular spasm (MVS) is still difficult due to the limited spatial resolution of existing intracoronary imaging devices, the development of MVS is generally identified by the reproduction of chest discomfort suggestive of angina with ischaemic ECG changes, in the absence of ≥90% epicardial stenosis during intracoronary ACh administration (Table 3).50
Almost a quarter of a century has passed since Mohri et al. originally described the incidence of MVS in a subset of patients with rest angina and normal epicardial coronary arteries.67 Indeed, it has been recently reported that the vast majority (97%) of INOCA patients with coronary functional abnormalities have epicardial or microvascular vasospasm in approximately equal proportions.68 Furthermore, in INOCA patients, MVS is sometimes provoked at lower doses of intracoronary ACh, followed by diffuse epicardial spasm at higher doses, indicating that a common mechanism is involved in both epicardial and microvascular spasms such as Rho-kinase activation.69
Meanwhile, for corroborating the entity of MVS, monitoring of myocardial lactate production throughout ACh provocation testing as an objective marker of myocardial ischaemia is recommended.27 Negative myocardial lactate extraction ratio, which is calculated as the ratio of the coronary arterial–venous difference in lactate concentration to the arterial concentration, is considered to be highly sensitive for myocardial ischaemia.69 Inevitably, MVS is defined as having a negative myocardial lactate extraction ratio despite the absence of angiographically demonstrable epicardial spasm throughout ACh provocation testing or prior to the occurrence of epicardial coronary spasm following intracoronary injection of ACh.67,69
Comprehensive Evaluation of Coronary Functional Abnormalities by Interventional Procedures
Recently, the combined invasive assessment of coronary vasoconstrictor and vasodilator abnormalities has been titled as an interventional diagnostic procedure (IDP), which typically consist of vasoreactivity testing with ACh and measurement of CFR and IMR with adenosine (Figure 2).12,70 Although there has been a critical missing link between IDP for assessment of coronary vasomotor function and health outcomes of INOCA patients, this gap has recently been addressed in the CorMicA randomised controlled trial.32,71 In this landmark study, Ford et al. demonstrated that an IDP linked to the stratified medication could ameliorate health status of patients with INOCA and that better quality of life was maintained among patients undergoing an IDP over 1 year.71 The IDP also allows us to acknowledge that several vascular dysfunction mechanisms may co-exist and overlap in a patient with INOCA.64,72 Furthermore, clarifying INOCA endotypes enable us to predict the clinical course of INOCA patients. The entity of impaired coronary microvascular dilator function predicts adverse cardiovascular outcomes, including death and non-fatal MI, in female INOCA patients, whereas enhanced epicardial coronary vasoconstrictive reactivity was related to angina hospitalisation.47 Additionally, in INOCA patients, coexistence of epicardial coronary spasm and increased microvascular resistance was associated with worse prognosis, for which Rho-kinase activation appears to be involved.64
Taken together, comprehensive assessment of coronary functional abnormalities with the IDP could be useful to develop a strategic and distinct outlook for managements of INOCA patients. This is the reason why the IDP is highly recommended for patients with chest pain and no obstructive CAD in the recent consensus documents on INOCA by the European Society of Cardiology.8 Meanwhile, CMD diagnosed based on the results of IDP may be associated with the presence of virtual histology intravascular-ultrasound-derived thin-cap fibroatheroma.73 Also, in our recent work, the prognostic links between coronary morphologies evaluated by optical coherence tomography and coronary vasomotor were noted in INOCA patients.74 These findings indicate that a combination of intracoronary imaging device-derived morphometric assessment and coronary functional assessment with IDP could greatly improve the prognostic prediction of INOCA patients.
Biomarkers of Coronary Functional Abnormalities
We have previously demonstrated that Rho-kinase activity in circulating neutrophils is a useful surrogate biomarker for coronary spasm, not only for the diagnosis of the disorder but also for the assessment of disease activity and efficacy of medical treatment.75 Furthermore, there is a circadian variation of Rho-kinase activity in circulating neutrophils with a peak noted in the early morning, associated with alterations in coronary basal tone and vasomotor reactivity.76
A previous experimental study showed that sustained elevation of serum cortisol level sensitises coronary VSMC to serotonin to cause coronary vasoconstrictive responses in pigs in vivo, suggesting the cross-link between stress and coronary artery spasm.77 In fact, Rho-kinase activity in circulating neutrophils in VSA patients was temporarily enhanced immediately after the Great East Japan Earthquake associated with disaster-related mental stress.78 Finally, Rho-kinase activity in circulating leucocytes is also useful for prognostic stratification of VSA patients.79 The combination of the JCSA risk score and Rho-kinase activity substantially improved risk stratification of VSA patients as compared with either alone.79 Taking these issues into consideration, Rho-kinase activity in circulating leucocytes appears to be a useful biomarker for coronary spasm with a broad versatility, comparing favourably with B-type natriuretic peptide in patients with heart failure and high-sensitivity cardiac troponin T or I in those with acute coronary syndrome.
Although the importance of CMD has been emerging, reliable biomarkers for CMD remain to be developed. Low-grade inflammation attracts much attention in the pathogenesis of CMD, since elevated C-reactive protein levels correlated with reduced CFR in patients with cardiac syndrome X, which is indicative of CMD.80 A recent study also demonstrated that cardiovascular protein biomarkers of inflammatory status and coagulation changes are associated with endothelium-independent CMD in female INOCA patients.81 Although several clinical studies previously addressed the relationship between systemic serotonin concentrations and coronary vasomotor abnormalities, we have recently demonstrated that plasma concentration of serotonin was significantly higher in patients with microvascular spasm as compared with controls.82 Importantly, in patients with INOCA, there was a positive correlation between plasma serotonin concentration and baseline thrombolysis in myocardial infarction frame count, a marker of coronary vascular resistance.11,82 These findings suggest that plasma concentration of serotonin may be a novel biomarker to predict latent microvascular spasm and enable us to dissect it from epicardial coronary artery spasm.
Conclusion
In summary, it is important to evaluate coronary vasomotor function comprehensively and diagnose coronary functional abnormalities including VSA and CMD precisely, particularly in INOCA patients. Further studies are needed to better understand the pathophysiology of coronary vasomotor dysfunction and to develop new effective therapeutic strategies for patients with coronary functional abnormalities.